Unveiling the Fascinating Fascial Network, Our Most Richly Sensory Organ
Dr. Robert Schleip
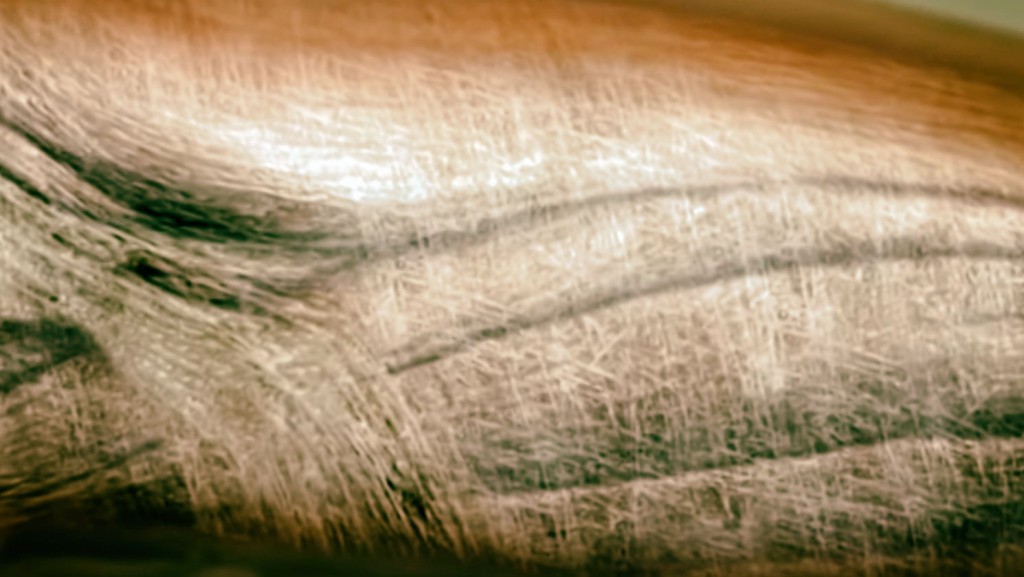
For decades, fascia has been viewed as a passive sheath providing mechanical support to our musculoskeletal system. While early reports hinted at the presence of sensory nerves in fascia, their significance was overlooked, leaving a gap in our understanding. Pioneers like Moshe Feldenkrais and Ida Rolf primarily attributed mechanical importance to fascia, unaware of its captivating sensory role. However, Andrew Taylor Still, the founder of osteopathy, recognized the presence of nerves in fascia, emphasizing their importance akin to branches of the brain.
In recent years, researchers like Jaap van der Wal have shed light on the intricate sensory nerve endings in muscle fascia, but conventional medicine largely disregarded these findings for decades. The turning point came with the first international Fascia Research Congress in 2007, where independent teams presented their breakthrough discoveries on fascial innervation. Subsequent studies emphasized the vital role of fascia in our body’s perception and internal representation.
German neurophysiologist Martin Grunwald estimated that the body-wide fascial network contains approximately 100 million nerve endings. A revised calculation, encompassing all fibrous collagenous connective tissues, suggests a staggering 250 million nerve endings, surpassing the sensory capacity of our eyes and skin. Fascia, constituting an average of 12.5 kg or 17.14% of total body weight, emerges as our richest sensory organ.
This newfound understanding prompts practitioners to explore the sensory qualities served by the dense innervation within fascia. The majority of receptors are interstitial, comprising thin neurons terminating in free nerve endings, sensitive to mechanical stimuli. Chemical or excessive mechanical stimulation can transform these receptors into nociceptors, signaling potential tissue damage. Notably, free nerve endings in the thoracolumbar fascia exhibit greater sensitivity to chemical stimuli than underlying muscles, leading to prolonged hypersensitivity.
These revelations challenge our perception of fascia, elevating it from a mere mechanical support system to a dynamic sensory entity. By unraveling the multifaceted nature of fascia, we embark on a journey of discovery, uncovering its profound impact on our body’s perception and potential therapeutic avenues.
Through continued research and collaboration, the enigmatic world of fascia unfolds, revolutionizing our understanding of the human body and opening doors to enhanced well-being and innovative treatment approaches.
Fascial Sensory Qualities and Receptors
The growing awareness of the rich sensory innervation within fascia has raised intriguing questions among practitioners. Figure 1 illustrates the key components, with interstitial receptors taking the spotlight. These receptors encompass neurons terminating in free nerve endings, including both C-fibers and Aδ-fibers. Remarkably thin, they exhibit heightened sensitivity to mechanical stimuli. When the extracellular matrix undergoes chemical alterations or the mechanical stimulation exceeds a threshold, these receptors can transform into nociceptors, signaling potential tissue damage to the central nervous system.
Notably, the mechanical activation threshold of free nerve endings in fascia is half that of the skin and muscles. Moreover, research reveals that free nerve endings in the human thoracolumbar fascia display significantly greater sensitivity to chemical stimuli compared to underlying muscles, leading to prolonged hypersensitivity.
It is vital for manual therapists to recognize that not all interstitial receptors are nociceptive. Some receptors serve thermoreception, highlighting the multifaceted nature of fascial sensory responses. The majority of interstitial receptors in fascial tissue are polymodal, meaning they respond to various types of stimulation.
As our understanding of these intricate receptors deepens, manual therapists gain valuable insights into the dynamic sensory landscape of fascia. By unraveling the complexities of fascial innervation, therapists can refine their approaches, optimizing therapeutic outcomes and empowering individuals on their path to well-being.
Enhancing Our Understanding of Fascial Receptors and Proprioception
There are four types of specialized proprioceptive mechanoreceptors in the fascia: Golgi receptors, Pacini receptors, Ruffini receptors, and muscle spindles.
The fascinating world of fascial receptors offers valuable insights into the mechanics of our bodies. Among them, Golgi receptors play a crucial role by adapting slowly and responding to tensile loads. Once thought to be exclusive to tendon tissue, these receptors have been discovered in various fascial tissues by independent researchers. Their abundance at myotendinous junctions and near intermuscular septa highlights their significance. When stimulated, Golgi receptors induce a relaxation response in the skeletal muscle fibers connected to tensioned collagen fibers. However, for stretching stimuli applied to extramuscular tendon tissue arranged in series with relaxed muscle fibers, the majority of the stretch is absorbed by the more compliant muscle fibers. To maximize effectiveness, it may be beneficial to combine stretching stimuli with brief muscle contractions or resistance. Manual transverse techniques that stretch fascial tissue orthogonally to adjacent muscle fibers, such as those by Tim Bowen, can elicit sufficient stretching of the passive collagen fibers despite the compliant muscle fibers in their surroundings.
Pacini receptors, on the other hand, adapt quickly and are particularly responsive to dynamic changes in mechanical stimulation. Their sensitivity makes gentle rocking and faster vibrations effective methods for stimulating these receptors, with potential positive effects on regional proprioception.
Another group of receptors, Ruffini receptors, adapt slowly and provide continuous sensory feedback. They convey long-lasting postural sensations and respond to “slow-melting” myofascial manipulation techniques. Notably, Ruffini receptors exhibit high sensitivity to shear stress, which supports the osteopathic approach of “local listening” through manual palpation to discern tissue movement preferences.
Among the specialized mechanoreceptors, muscle spindles are fusiform structures surrounded by a dense connective tissue capsule. While absent in fish, muscle spindles are found in humans, particularly in muscles involved in opposing gravity. The discovery of Piezo2 membrane receptors on the surface of human muscle spindles has garnered significant attention since the 2021 Nobel Prize in Physiology or Medicine. These small yet highly sensitive membrane receptors play a crucial role in proprioception. Studies have shown reduced expression of Piezo2 receptors in individuals with adolescent idiopathic scoliosis, leading to impaired proprioception. Additionally, muscle spindles in individuals with scoliosis are noticeably smaller than those in healthy individuals. These findings have sparked excitement among therapists and researchers studying scoliosis.
Spindle capsules are typically embedded in the intramuscular fascial layer of the perimysium. The stiffness of the surrounding perimysium is believed to impact the sensitivity of muscle spindles, as they require tension differences of at least 3 g to detect changes. Increased stiffness associated with chronic immobilization and fibrotic pathologies can negatively affect proprioception, similar to genetically determined differences in muscle spindle expression in adolescent idiopathic scoliosis.
By unraveling the complexities of these fascial receptors and their role in proprioception, we gain valuable insights that can enhance therapeutic approaches and our understanding of the intricate mechanics of the human body.
Fascial Interoception
The term interoception refers to bodily perceptions that are related to the body’s search for homeostasis and physiological needs, rather than its orientation in space. Interoceptive signaling encompasses somatic perceptions such as temperature changes, hunger, thirst, nausea, tingling/flowing sensations, muscle soreness, oxygen supply, muscle exertion, and a sense of belonging or alienation in specific body regions. These perceptions are mediated by free nerve endings, known as interstitial receptors, located in visceral connective tissue and within perimysial and endomysial fascial tissues.
The neural pathways for interoceptive stimulation differ from those for somatic sensations. Instead of projecting to the somatomotor cortex, interoceptive neurons project to the insular cortex (insula), a folded region of cortical gray matter within the forebrain. In the insula, internal somatic sensations are linked to emotional preferences, expectations, and feelings. Impaired insular function can lead to preserved biomechanical function and high cognitive test scores but may result in impaired social abilities and difficulties in making reasonable decisions in complex situations.
Impaired proprioceptive perception is associated with various conditions such as back pain, scoliosis, and complex regional pain syndrome. On the other hand, dysfunctional interoceptive processing is more closely linked to disorders such as eating disorders, anxiety disorders, depression, irritable bowel syndrome, obsessive-compulsive disorder, alexithymia, and post-traumatic stress disorder (see Table 1).
Therapists should reconsider their typical questions during massage or bodywork to shift the focus of their patients towards proprioceptive or interoceptive perceptions. Relying solely on questions about body orientation may limit therapeutic improvement if a more interoceptive approach is needed to refine perception. Skillfully promoting visceral fascial sensations through gentle visceral manipulations or directing attention to potential sensations of flow, energy, or temperature changes in specific body regions can have more profound effects than solely focusing on musculoskeletal sensations.
Table 1: Different Pathologies and Their Associated Impairments of Proprioception and Interoception
Proprioceptive Impairment | Interoceptive Dysregulation |
---|---|
Back Pain | Eating Disorders, Irritable Bowel Syndrome |
Whiplash Injury | Post-Traumatic Stress Disorder |
Complex Regional Pain Syndrome | Substance Abuse, Addiction Disorders |
Attention-Deficit/Hyperactivity Disorder (ADHD) | Depression, (Generalized) Anxiety Disorder |
Adolescent Idiopathic Scoliosis | Autism Spectrum Disorders, Depersonalization/Derealization Disorder |
Systemic Hypermobility | Somatic Symptom Disorders, Functional Disorders |
Other Myofascial Pain Syndromes | Fibromyalgia, Chronic Fatigue Syndrome |
Fascia as a Source of Pain
Studies investigating fascial nociception have primarily focused on the thoracolumbar fascia, which has been found to be densely innervated with free nerve endings that transmit nociceptive signals to neurons in the spinal cord’s dorsal horn. Stimulation of the thoracolumbar fascia, such as through the injection of mildly irritating substances, elicits stronger and longer-lasting pain sensations compared to stimulation of underlying muscles. Patients experiencing fascial pain often describe it as burning, throbbing, or stabbing.
Delayed onset muscle soreness (DOMS), characterized by a decreased pain threshold in muscle tissue after intense exertion, is largely attributed to hypersensitivity of the fascial sheaths surrounding the involved muscles. Following the induction of DOMS in experiments, the pain threshold in the fascia decreases more significantly than in the underlying muscle tissue. These findings suggest that fascial tissue plays a role in transmitting nociceptive stimuli or irritations to the spinal cord. However, the perception of pain resulting from nociceptive stimulation depends on various factors, including individual cortically driven threat processing. The tissue origin often makes a significant difference, as the central nervous system tends to respond particularly sensitively to fascial nociception compared to muscular nociception.
Therapists trained in neurophysiology often rely on the gate control theory to explain the pain-reducing effects of their work. However, recent advancements in pain research have shown that this model is only partially correct. Proprioceptive signals can have analgesic effects within their specific innervation regions in the spinal cord, and the speed of incoming neuronal stimulation does not appear to be crucial for this effect.
Recent investigations have shown that polymodal receptors in the spinal cord’s dorsal horn have a strong demand for stimulation from their respective peripheral innervation areas. These synapses seem to be easily satisfied when sufficient proprioceptive information is supplied through polymodal receptors in these tissue regions. However, when there are stiffenings in the connective tissue matrix surrounding the receptors, interstitial neurons actively lower their threshold for nociceptive stimuli, leading to myofascial pain. Additionally, they can release cytokines that sensitize polymodal neurons in their peripheral innervation regions, predisposing these areas to nociceptive function. Even minor mechanical stimulations, such as a slight difference in leg length or wear in the jaw joint, may potentially trigger nociceptive responses within the complex network of intrafascial polymodal receptors.
Autonomic Nervous System and Fascial Stiffness
Recently, extensive histological investigations have revealed sympathetic nerve endings to be the most common receptor type in human fascia. These nerve endings account for approximately 40% of the total fascial innervation. They have a vasoconstrictive effect and play a crucial role in controlling blood flow, including microcirculation through arterioles and venules. The numerous sympathetic nerve endings in the fascia influence regional changes in tissue temperature, supply of oxygen and nutrients, fluid flow, and removal of waste products. They significantly contribute to the warmth or coldness of tissue regions and the accumulation of inflammatory cytokines and free radicals due to stagnation of fluid flow. The body-wide fascial network contains approximately 100 million sympathetic nerve endings.
Psychoneuroimmunology explores the influence of emotions on the autonomic nervous system and the immune system. Combined with the extensive sympathetic control of microcirculation, subtle changes in skin temperature often reflect acute and chronic emotional states. Thermography is increasingly used in psychological research to investigate this profound interaction. In manual therapies, practitioners with sufficient training can also gather precise information by sensing changes in skin temperature with their hands.
Interestingly, not all sympathetic nerve endings in the fascia are located near blood vessels. Some of them appear to have a trophic function, influencing the biochemical milieu of their environment.
German physiologist Jochen Staubesand discovered contractile cells with morphological features similar to smooth muscle in the dense fascia of the human lower leg, along with a rich presence of sympathetic nerve endings. He proposed a close connection between chronic sympathetic activation and fascial stiffness, suggesting that myofascial tone adjustments are regulated through two different systems. While muscle fibers quickly adapt their active contractile behavior under the control of the somatic nervous system, the stiffness of fascial tissue elements is regulated by the autonomic nervous system, resulting in slower and longer-lasting changes in tonicity. Chronic emotional stress and other psychoemotional health manifestations play an important role in this process. Staubesand emphasized that any intervention in the fascia affects the autonomic system as a whole and all organs directly influenced by it:
“It now appears that fascial tone is influenced and regulated by the state of the autonomic nervous system. Furthermore—and this should have implications for your work—any intervention in the fascial system also affects the autonomic system as a whole, as well as all organs directly influenced by the autonomic nervous system. To put it simply, any intervention in the fascia is also an intervention in the autonomic system”.
This fascinating new hypothesis motivated our small research group at the University of Ulm to continue investigating the active contractile capacity of fascial tissue through extensive laboratory studies over a period of 10 years.
In our final publications, we documented the presence of contractile cells in all the examined fasciae, although with differences in their density.
Interestingly, the human thoracolumbar fascia showed a higher density of such cells compared to other human fasciae or the lumbar fascia of the animals we studied. These contractile cells are myofibroblasts, an intermediate form between fibroblasts (connective tissue cells) and smooth muscle cells, possessing characteristics of smooth muscle cells [30], [31]. Such cells have been observed in wound healing as well as in fibrotic pathologies such as frozen shoulder or Dupuytren’s contracture.
When we examined the contractile response of different fascial tissues to chemical stimulation (using small tissue samples suspended in a pre-stretched state in physiological solution), we observed a significant stiffening response to various cytokines, particularly thromboxane and TGF-β1 (a cytokine associated with tissue fibrosis and wound healing). In the context of minutes or hours, the speed and magnitude of the contractile response were ridiculously low. This seemed to correspond with the reported slow contraction speed of fibrotic pathologies (such as burn scars or frozen shoulder) that contract at a rate of approximately 1 cm per month.
At Staubesand’s suggestion, our group investigated whether stimulating the fasciae with adrenaline or other synaptic transmitters of the autonomic nervous system could induce a contractile response. Contrary to his and our expectations, this was not the case: our experimental setup did not result in a clear stiffening response to adrenaline, acetylcholine, etc.
Years later, we learned of a new finding: in psychoneuroimmunology, it has long been known that chronic sympathetic stress activation can lead to altered activation of T3 immune cells in lymph nodes. This functional influence is often observed when people are unusually resistant to infections in the last days of intense work-related stress but become more susceptible to infections in the initial days of recovery. For a long time, the missing link or specific cytokine between sympathetic activation and the immune reaction was not kn
Recently, extensive histological investigations led by Siegfried Mense’s team from the University of Heidelberg have revealed that sympathetic nerve endings are the most prevalent receptor type in human fascia. These receptors, accounting for approximately 40% of the total fascial innervation, have a vasoconstrictive effect and play a crucial role in controlling blood flow and microcirculation. The sympathetic nerve endings in the fascia influence tissue temperature, oxygen and nutrient supply, fluid flow, and the removal of waste products. They are responsible for warmth or coldness in tissue regions and the accumulation of inflammatory cytokines and free radicals. Approximately 100 million sympathetic nerve endings are present in the body-wide fascial network.
The field of psychoneuroimmunology explores the influence of emotions on the autonomic nervous system and the immune system. The extensive control of microcirculation by the sympathetic system explains how subtle changes in skin temperature can reflect acute and chronic emotional states. In manual therapies, skilled practitioners can detect these changes through their hands’ sensitivity to skin temperature.
Notably, a significant portion of sympathetic nerve endings in the fascia is not located near blood vessels. These nerve endings appear to have a trophic function, influencing the biochemical environment of their surroundings.
German physiologist Jochen Staubesand discovered contractile cells resembling smooth muscle in the dense fascia of the lower leg, along with abundant sympathetic nerve endings. He proposed a close connection between chronic sympathetic activation and fascial stiffness, suggesting that the autonomic nervous system regulates myofascial tone adjustments. This differs from the somatic nervous system’s control of muscle fibers. Chronic emotional stress and psychoemotional health manifestations play a role in this process. Staubesand emphasized that any intervention in the fascia also affects the autonomic system and the organs directly influenced by it.
A research group at the University of Ulm conducted extensive laboratory studies over a period of 10 years, documenting the presence of contractile cells called myofibroblasts in different fascial tissues. The density of these cells was found to be higher in the human thoracolumbar fascia compared to other fascial tissues. When stimulated with cytokines such as thromboxane and TGF-β1, the fascial tissues displayed a significant stiffening response. However, in their experimental setup, the contractile response of fascial tissues to adrenaline or other synaptic transmitters of the autonomic nervous system did not produce a clear stiffening response.
Further research in psychoneuroimmunology has revealed that chronic activation of the sympathetic stress response can affect T3 immune cells in lymph nodes, leading to altered immune reactions. Surprisingly, the cytokine TGF-β1, which triggers tissue stiffening, was identified as the missing link between sympathetic activation and immune response. Fibroblasts are responsible for producing and depositing TGF-β1 in specific pockets within the surrounding ground substance. Under conditions of significant mechanical or biochemical stress, these pockets open, allowing the fibroblasts to transform into myofibroblasts with enhanced contractile force. This transformation occurs within a few hours, similar to the fictional character Asterix’s transformation after consuming his magic potion. Experimental tissue contraction tests revealed that contractions in fascial tissues with a higher density of myofibroblasts were significantly stronger.
In another laboratory setting, contractile cells were kept alive for several days and stimulated with the hormone adrenaline. Colleagues in Taiwan discovered that fibroblasts did not immediately increase their production of TGF-β1, but rather exhibited a time delay of approximately 24 hours. This finding highlighted that the observation time in previous laboratory experiments on tissue contraction was insufficient to detect a clear stiffening response to adrenaline stimulation. However, the most important finding was that chronic sympathetic activation can indeed induce a stiffening response in fascial tissues through altered expression of adrenaline. This process, however, occurs over a slower and more enduring timeframe rather than within a few hours.
Robert Schleip’s Fascinating Fascia
Are you ready to take your myofascial treatment to the next level? Don’t miss this incredible opportunity to learn from one of the foremost experts in the field—Dr. Robert Schleip!
In his captivating presentation, Dr. Schleip will unveil the most important insights from fascia research and demonstrate their practical application in myofascial treatment.
* Explore the Fascinating Connection Between Fascia and the Autonomic Nervous System: Discover how the intricate relationship between fascia and the autonomic nervous system affects musculoskeletal issues. Gain a deeper understanding of the underlying mechanisms and uncover valuable implications for enhancing treatment outcomes.
* Delve into the Emotional Dimensions of Fascia: Uncover the profound connection between fascia and emotions. Dr. Schleip will share groundbreaking research findings that shed light on how addressing the fascial system can positively impact conditions such as post-traumatic stress disorder (PTSD). Expand your treatment possibilities and offer new hope to those suffering from musculoskeletal and emotional issues.
* Practical Application of Research Findings: Witness Dr. Schleip’s live demonstrations of practical techniques derived from his extensive research. Learn how to directly apply these findings to optimize your myofascial treatment protocols. Elevate your skills and revolutionize your practice!
Sydney 27-29 October 2023. Register at:
https://terrarosa.com.au/product-category/hands-on-workshops/robert-schleips-fasciniating-fascia/